Research
GENERAL RESEARCH INTERESTS
Solid-state NMR spectroscopy, oxygen-17 and oxygen-18 isotopic labeling, mechanochemistry, functional biomaterials and (nano)materials, structure and reactivity of interfaces, operando techniques for ball-milling, combined experimental-computational approaches in NMR, quadrupolar nuclei, isotope effects.
OVERALL GOAL
The objective of the MISOTOP project is to develop a better understanding of the structure and properties of a variety of functional (nano)materials, thanks to advanced spectroscopic analyses involving the stable isotopes of oxygen (especially oxygen-17 NMR). Investigations are performed along 4 main directions :
- The implementation of new 17O and 18O isotopic labeling protocols using mechanochemistry
- Advanced NMR studies on 17O-enriched compounds
- The study of oxygen bonding environments at interfaces
- The implementation of new operando methods for elucidating reaction mechanisms in ball-milling
More details on these studies can be found below and in the associated publications.
1. Oxygen-17 and Oxygen-18 isotopic labeling using mechanochemistry
Because of the very low natural abundance of oxygen-17 (0.037%) and oxygen-18 (0.20 %), isotopic labeling is often needed for performing high-resolution spectroscopic studies of molecules and materials. However, up until recently, most oxygen-enrichment schemes were simply unattractive to a broad research community, because they were costly (due to the use of excessive quantities of enriched precursors) and/or experimentally constraining (due to the long reaction times or harsh synthetic conditions). This implied that spectroscopic techniques such as 17O NMR were only used by a small number of research groups.
In 2017, we demonstrated for the first time that synthetic protocols based on ball-milling can be used for the 17O-labeling of organic and inorganic compounds in a cost-efficient and user-friendly way. The reactions are all performed in a few hours under ambient conditions, and using very small quantities of 17O (or 18O) enriched water. Since then, we have been expanding the families of compounds which can be labeled by mechanochemistry, showing that it is applicable to oxides (SiO2, Al2O3, TiO2, ZrO2…), hydroxides (Ca(OH)2, Mg(OH)2…), carboxylic ligands (terephthalic acid, trimesic acid…), fatty acids (stearic acid, oleic acid…), and other small molecules of biological or pharmaceutical interest (ibuprofen…). Moreover, we have started to work on the scale-up of some of these reactions, so that the 17O (or 18O) enriched species can then be used as precursors in standard synthetic protocols of molecules and materials, and thereby help answer the needs of a wide community of chemists.
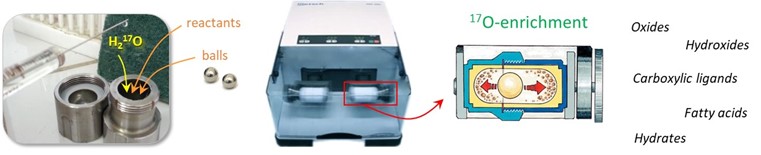
2. Advanced NMR studies on 17O-enriched compounds
In this context, we have been working along several directions, which include (i) performing higher resolution analyses at ultra-high fields (20 to 35.2T) to help finely resolve different oxygen sites, (ii) studying the structure and dynamics of various oxygen bonding environments through variable temperature analyses and computational modeling (including ab initio calculations of NMR parameters and molecular dynamics simulations), and (iii) using dynamic nuclear polarization (DNP) techniques for better resolving some of the 17O-sites enriched in ball-milling. Some of these investigations are performed on the instruments available in large scale facilities (Infranalytics network in France, UK high field NMR facility in Warwick, MagLab in Tallahassee), and in close collaboration with researchers from these laboratories.
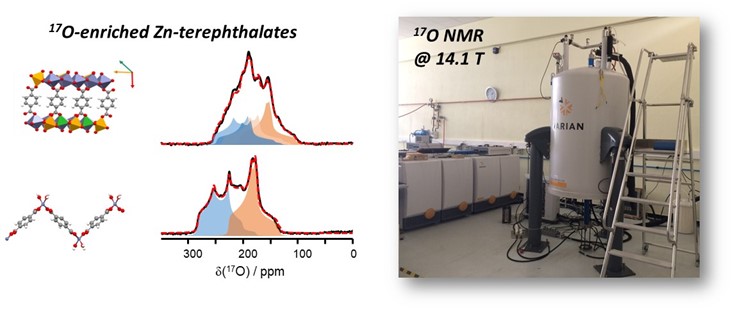
3. Elucidation of interfacial bonding environments
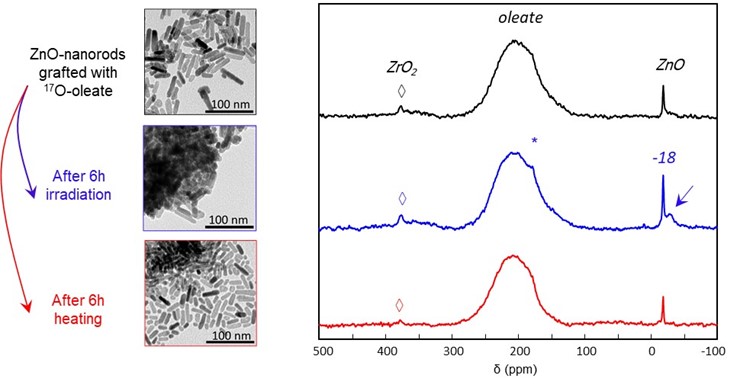
4. Understanding reaction mechanisms in ball-milling
While the use of ball-milling is drawing increasing attention for the synthesis of functional molecules and materials, one of the main goals of the mechanochemistry community is now to gain deeper knowledge into the actual reaction mechanisms occuring inside the milling jars. This can be done through ex-situ analyses of reaction media at different time points (using techniques like IR spectroscopy, powder X-ray diffraction, or solid state NMR, including 17O-NMR). However, some reaction intermediates cannot be observed through ex-situ analyses, which explains why much effort is being put into the development of operando analytical methods, including synchrotron powder X-ray diffraction and Raman spectroscopy.
In Montpellier, we have started to couple different operando analytical methods (Raman analyses, thermal camera measurements…), some of which had never been proposed before (acoustic analyses). We have shown that this can not only help understand the formation of coordination networks or co-crystals under ball-milling conditions, but also help rationalize some of the changes in physical aspect of the reaction media during ball milling
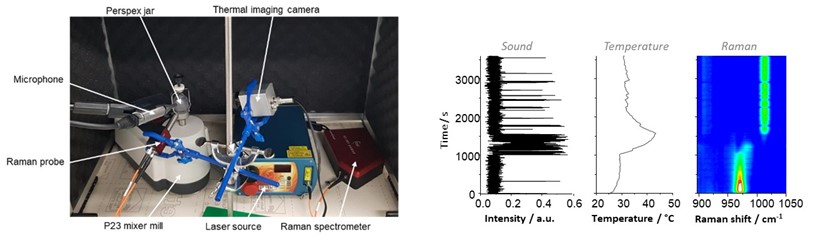